2025
“DFT+U Calculations on Substitutionally Doped (Ni, Cu, Zn) Mg-vanadate Surfaces for the Oxidative Dehydrogenation of Alkanes”
Submitted.
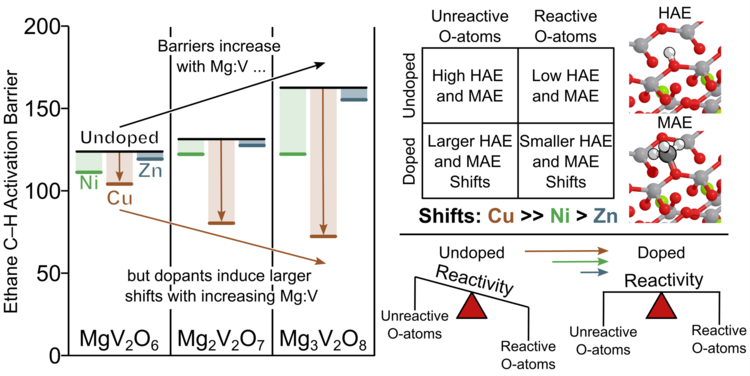
Abstract
“Modified adsorption energies on single-layer IrO2 and RuO2 films of IrO2-RuO2 heterostructures: Localized effect of subsurface metal-oxygen ligands”
Submitted.
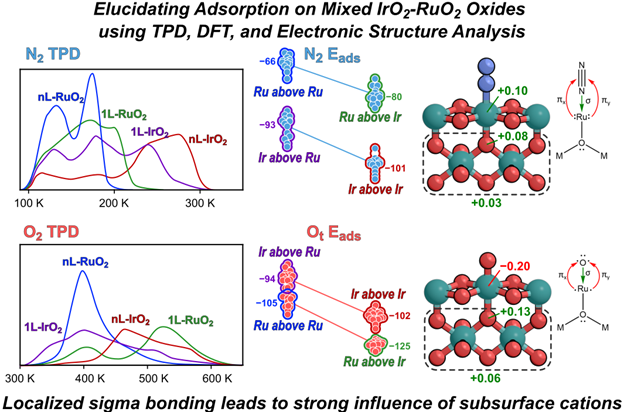
Abstract
“Predicting a Generalized Mechanism of Branched Hydrogenolysis on Ru, Ir, and Pt Surfaces for Polymer Upcycling Applications”
Submitted.
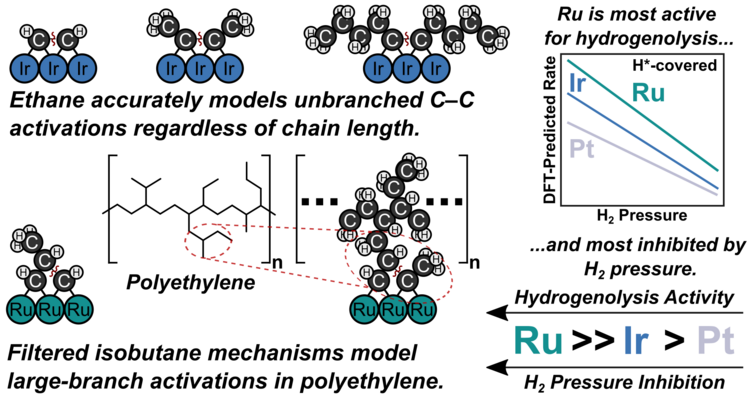
Abstract
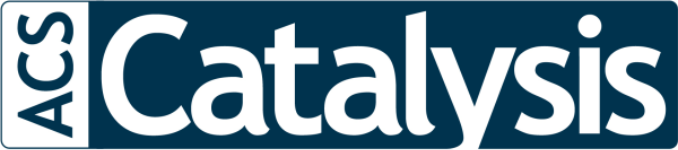
“Assessing the Influence of Void Environment in MFI Zeolites on Propene Oligomerization Kinetics Using a Combined Computational and Experimental Approach”
ACS Catalysis, 15 (2025) 7121–7137.
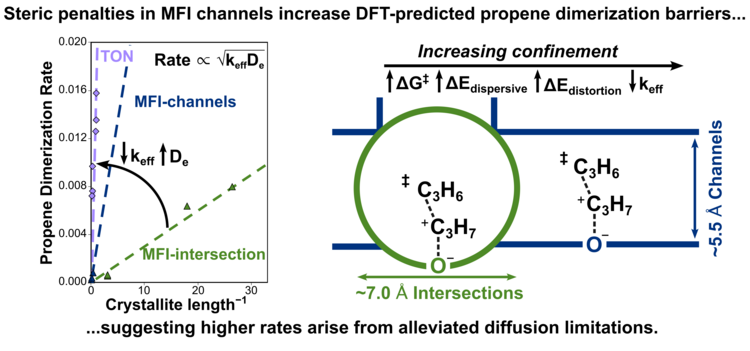
Abstract
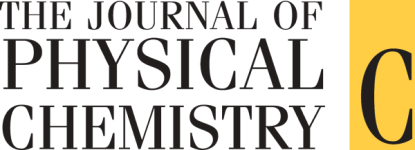
“Understanding AuPd Alloy Nanoparticle Structure under Vacuum Using DFT and Monte Carlo Methods”
Journal of Physical Chemistry C, 129 (2025), 5702–5717.
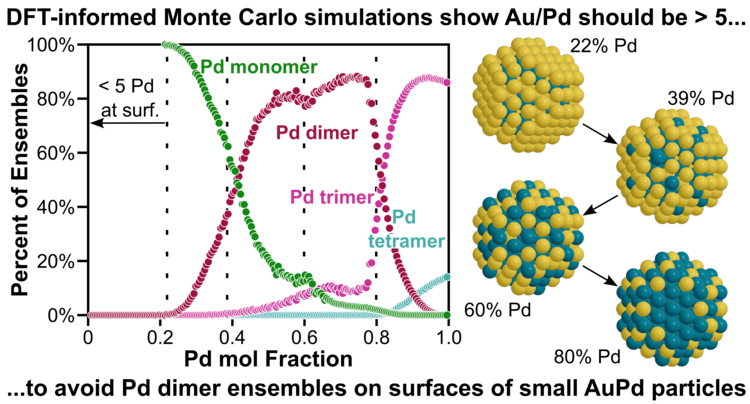
Abstract
2024
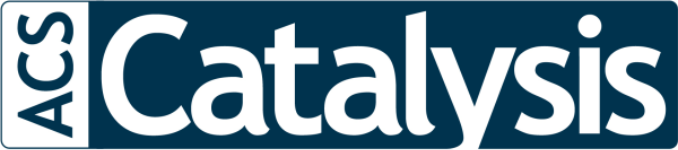
“A Career in Catalysis: Enrique Iglesia”
ACS Catalysis, 14 (2024) 10583–10613.
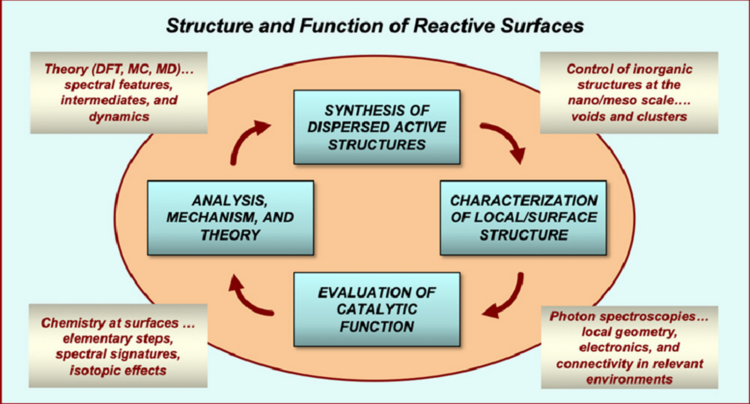
Abstract
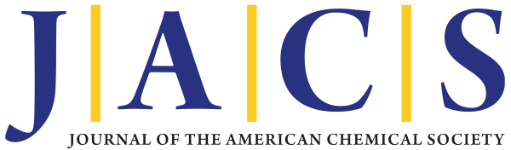
“Synthetic Placement of Active Sites in MFI Zeolites for Selective Toluene Methylation to para-Xylene”
Journal of the American Chemical Society, 146 (2024) 10666–10678.
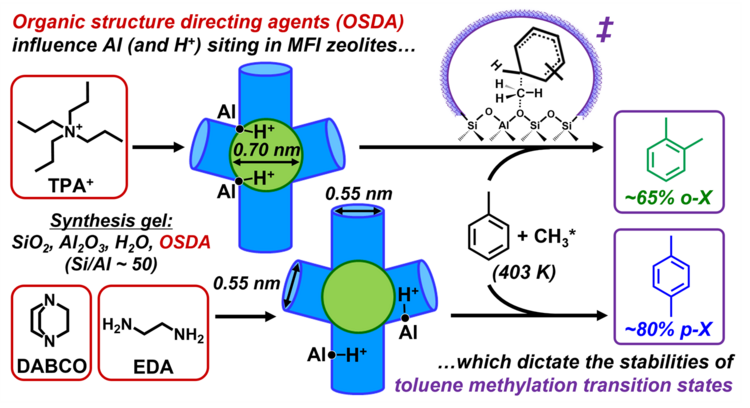
Abstract
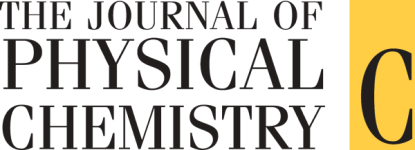
“Predicting the Enthalpy of Hydrocarbon Radicals Adsorbed on Pt(111) Using Molecular Fingerprints and Machine Learning”
Journal of Physical Chemistry C, 128 (2024) 5030–5043.
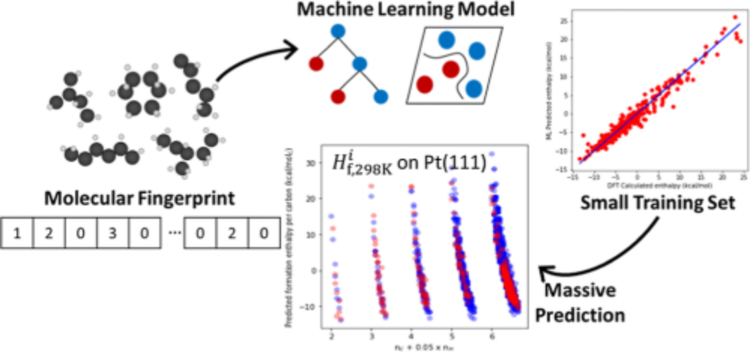
Abstract
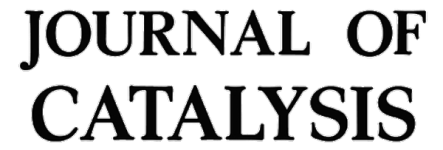
“Kinetics and Reaction Mechanism of Pd-Catalyzed Chlorobenzene Hydrogenolysis”
Journal of Catalysis, 432 (2024) 115435.
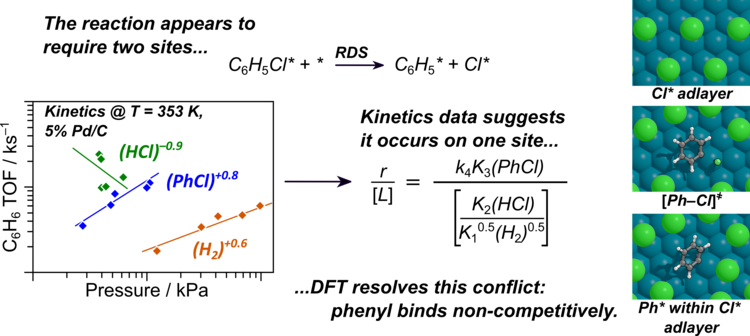
Abstract
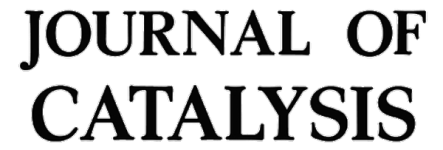
“Electronic and Geometric Features Controlling the Reactivity of Mg-vanadate and V2O5 Surfaces toward the Initial C–H Activation of C1–C3 Alkanes – a DFT+U Study”
Journal of Catalysis, 442 (2025) 115800.
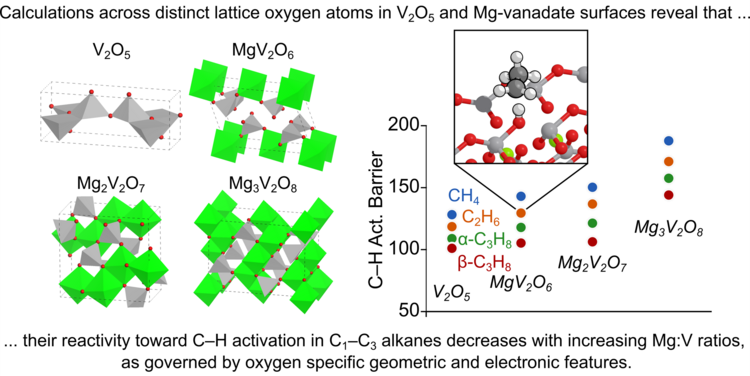
Abstract
2023
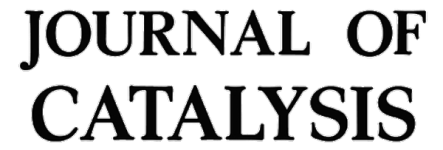
“Role of Phosphorous in Transition Metal Phosphides for Selective Hydrogenolysis of Hindered C–O Bonds”
Journal of Catalysis, 421 (2023) 403–418.
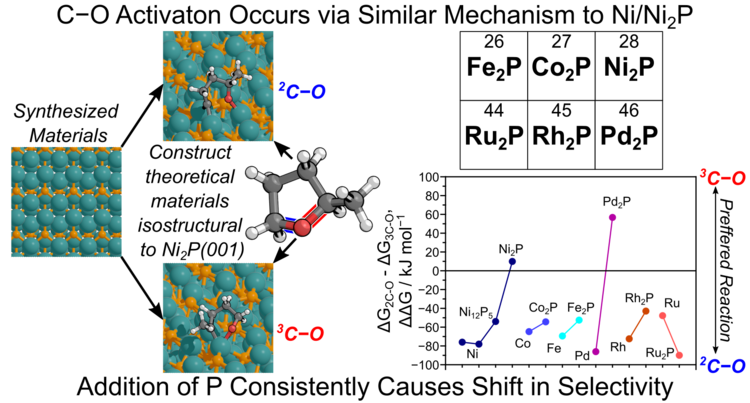
Abstract
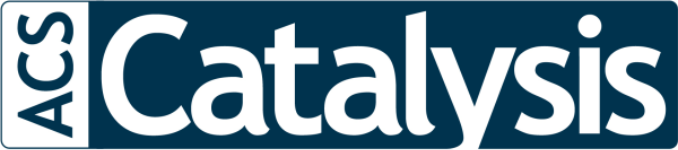
“Brønsted Acid Strength Does Not Change for Bulk and External Sites of MFI”
ACS Catalysis, 13 (2023) 4470–4487.
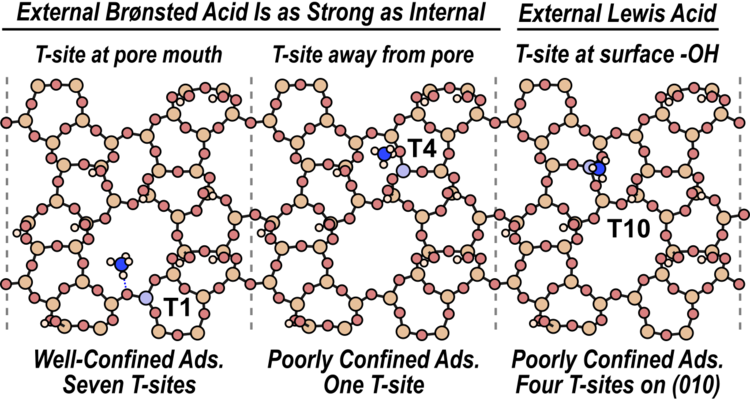
Abstract
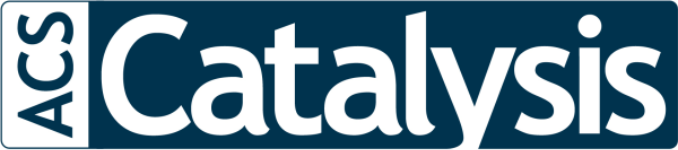
“Mechanisms and Kinetics of the Dehydrogenation of C6-C8 Cycloalkanes, Cycloalkenes, and Cyclodienes to Aromatics in H-MFI Zeolite Framework”
ACS Catalysis, 13 (2023) 99–112.
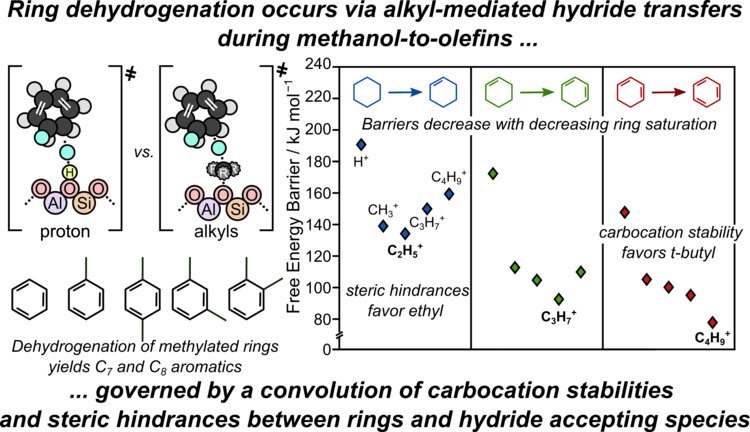
Abstract
2022
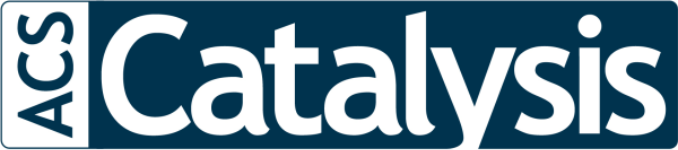
“Experimental and theoretical characterization of Rh single-atoms supported on γ-Al2O3 with varying hydroxyl content during NO reduction by CO”
ACS Catalysis, 12 (2022) 11697–11715.
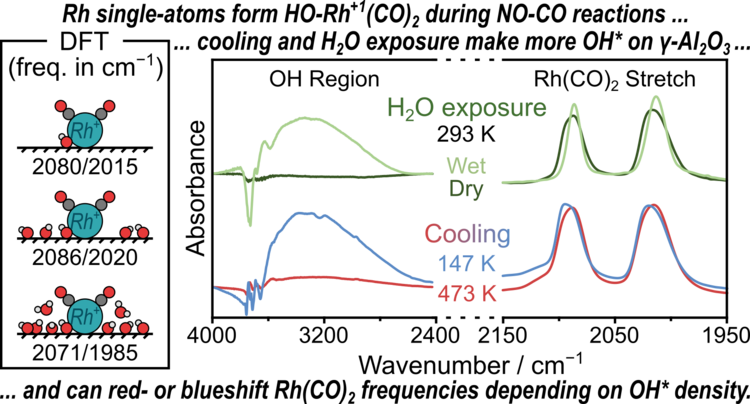
Abstract
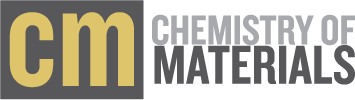
“Altering the Arrangement of Framework Al Atoms in MEL Zeolites Using Mixtures of Tetrabutylammonium and Sodium Structure-Directing Agents”
Chemistry of Materials, 34 (2022) 6835–6852.
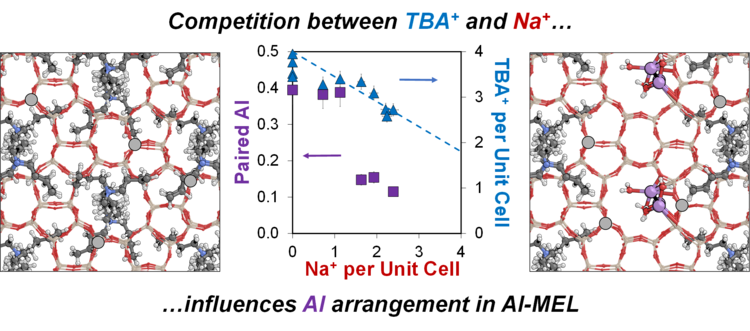
Abstract
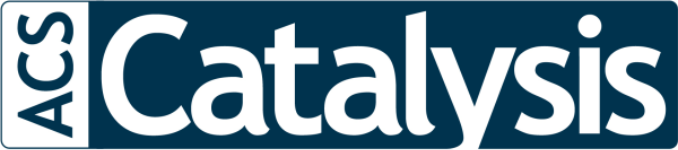
“Oxygen-Doped Carbon Supports Modulate the Hydrogenation Activity of Palladium Nanoparticles through Electronic Metal−Support Interactions”
ACS Catalysis, 12 (2022) 7344–7356.
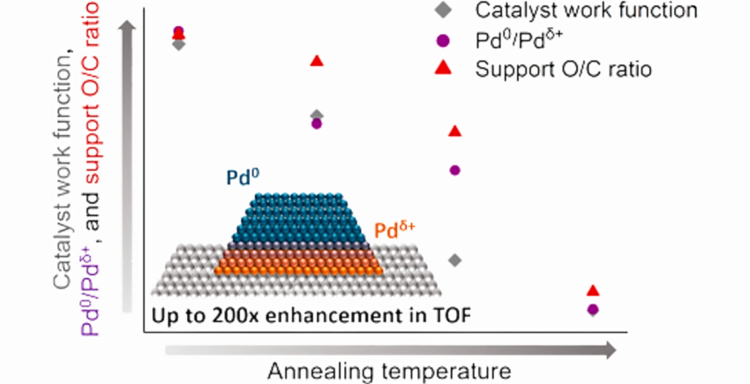
Abstract
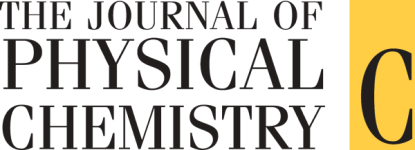
“Binding and Exchange Reactions of Hydrogen Isotopes on Surfaces of Dispersed Pt Nanoparticles”
Journal of Physical Chemistry C, 126 (2022), 3923–3938.
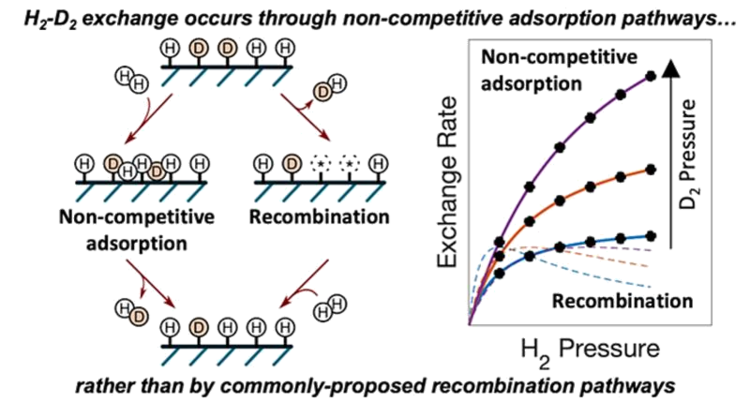
Abstract
“Predicting diffusion barriers and diffusivities of C6–C12 methylbenzenes in MFI zeolites”
Microporous and Mesoporous Materials, 333 (2022), 111705.
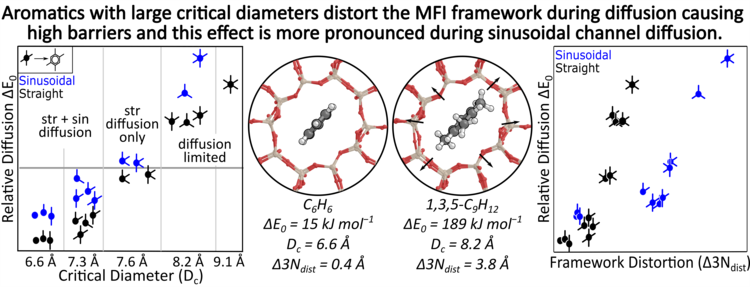
Abstract
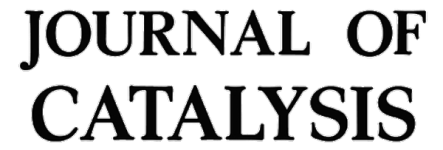
“The Fischer-Tropsch synthesis: A few enduring mechanistic conundrums revisited”
Journal of Catalysis, 405 (2022) 614–625. Perspective

First Paragraph
2021
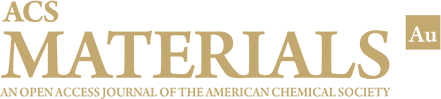
“Quantifying effects of active site proximity on rates of methanol dehydration to dimethyl ether over CHA zeolites through microkinetic modeling”
ACS Materials Au, 2 (2021), 163–175.
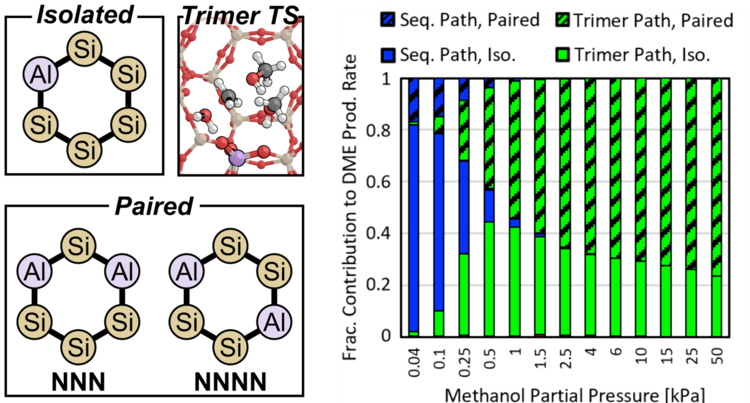
Abstract
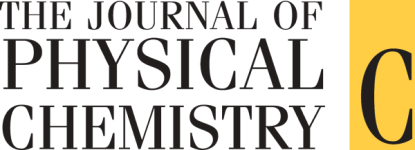
“Theoretical and experimental characterization of adsorbed CO and NO on γ-Al2O3-supported Rh nanoparticles”
Journal of Physical Chemistry C, 125 (2021) 19733–19755.
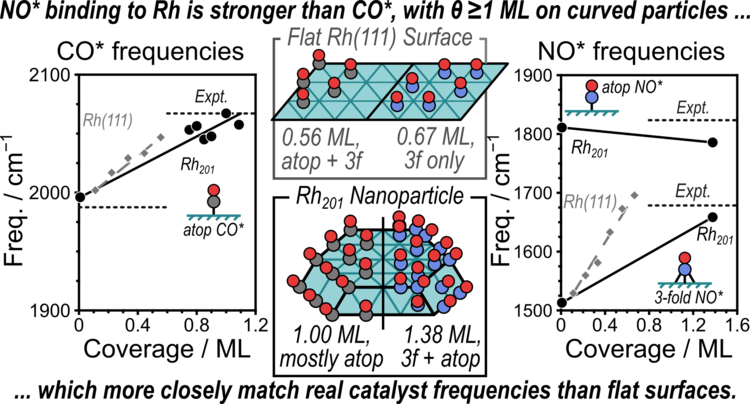
Abstract
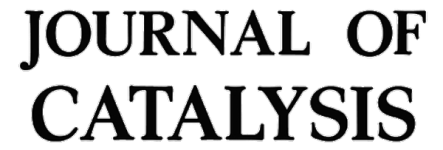
“Comparing Alkene Disproportionation and Formaldehyde-mediated Diene Formation Routes in Methanol-to-Olefins Catalysis in MFI and CHA”
Journal of Catalysis, 400 (2021) 124–139.
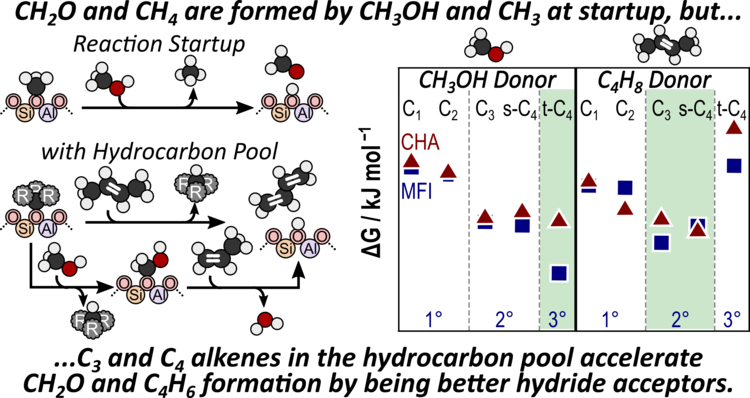
Abstract
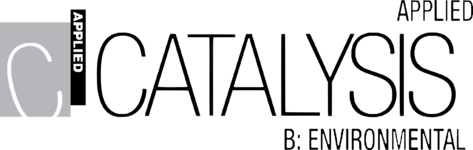
“Hydrogenation and C-S bond activation pathways in thiophene and tetrahydrothiophene reactions on sulfur-passivated surfaces of Ru, Pt, and Re nanoparticles”
Applied Catalysis B: Environmental , 291 (2021) 119797.
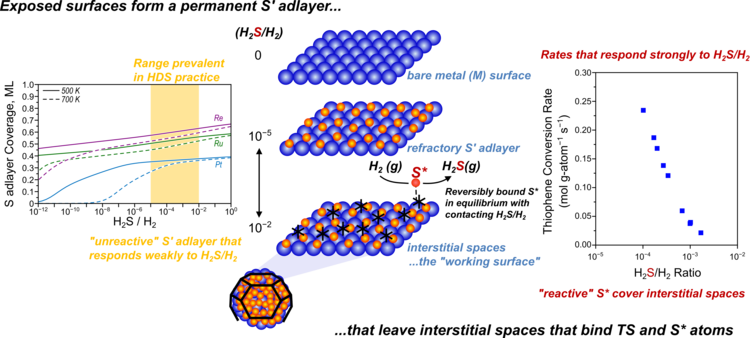
Abstract
2020
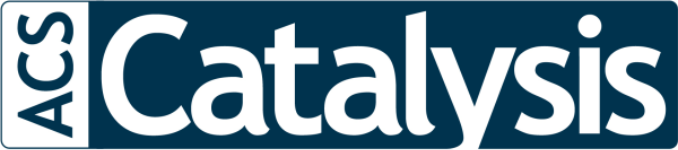
“Oxophilicity Drives Oxygen Transfer at a Palladium-Silver Interface for Increased CO Oxidation Activity”
ACS Catalysis, 10 (2020) 13878–13889.
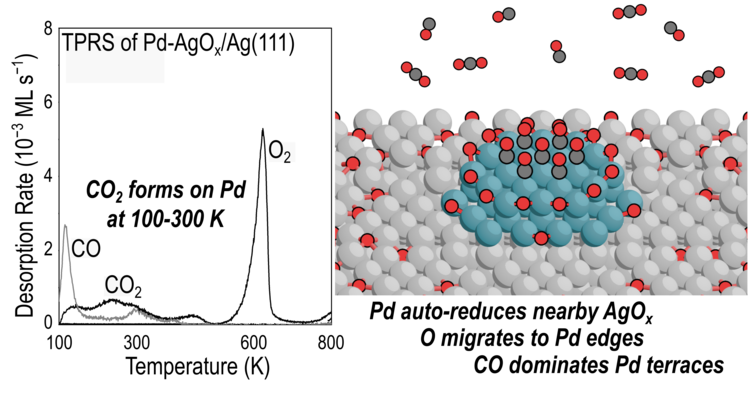
Abstract
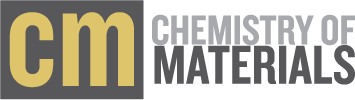
“Experimental and Theoretical Assessments of Aluminum Proximity in MFI Zeolites and its Alteration by Organic and Inorganic Structure-Directing Agents”
Chemistry of Materials, 32 (2020) 9277–9298.
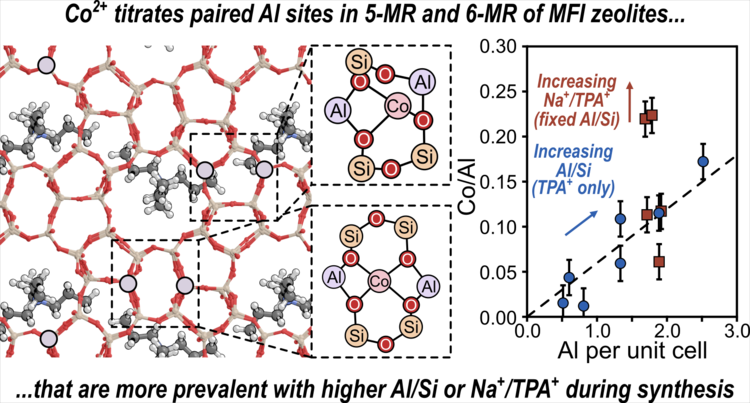
Abstract
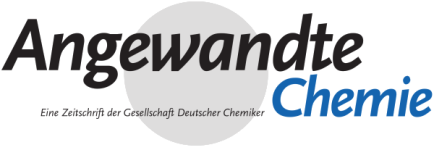
“Rigid Arrangements of Ionic Charge in Zeolite Frameworks Conferred by Specific Al Distributions Preferentially Stabilize Alkanol Dehydration Transition States”
Angewandte Chemie Int. Ed., 59 (2020) 18686–18694.
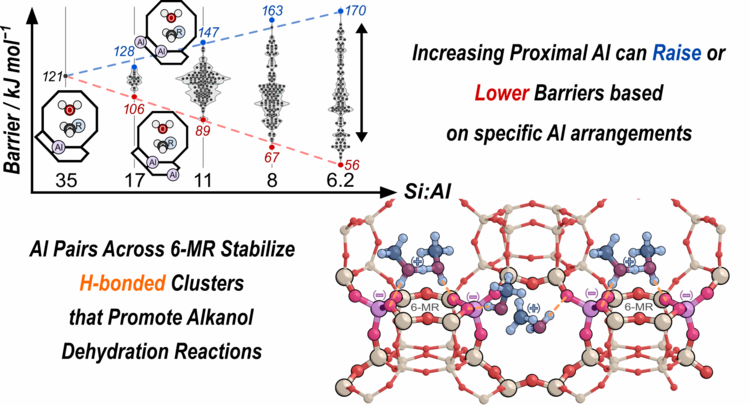
Abstract
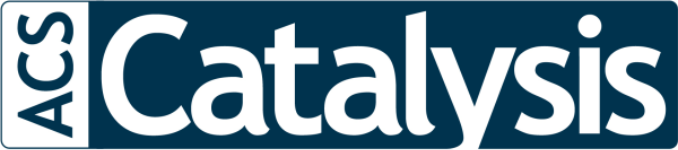
“Highly Selective Cross-Etherification of 5-Hydroxymethylfurfural with Ethanol”
ACS Catalysis, 10 (2020) 6771–6785.
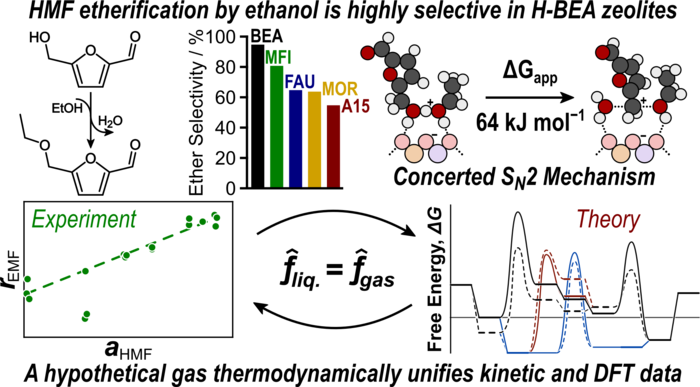
Abstract
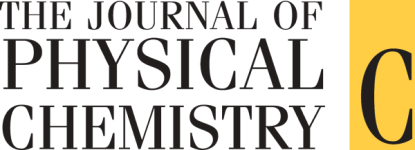
“Mechanism and Effects of Coverage and Particle Morphology on Rh and Pt Catalyzed NO-H2 Reactions”
Journal of Physical Chemistry C, 124 (2020) 13291–13303.
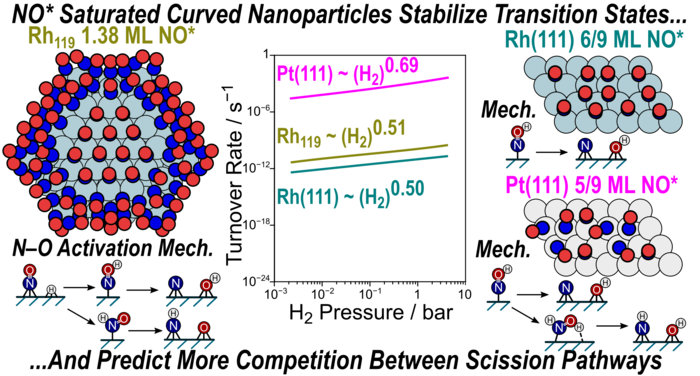
Abstract
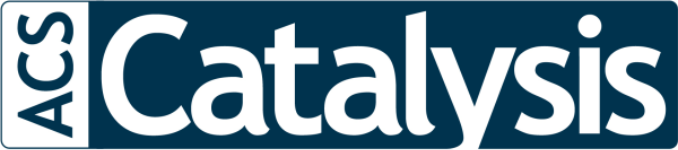
“Contrasting Arene, Alkene, Diene, and Formaldehyde Hydrogenation in H-ZSM-5, H-SSZ-13, and H-SAPO-34 Zeolite Frameworks during MTO”
ACS Catalysis, 10 (2020) 4593–4607.
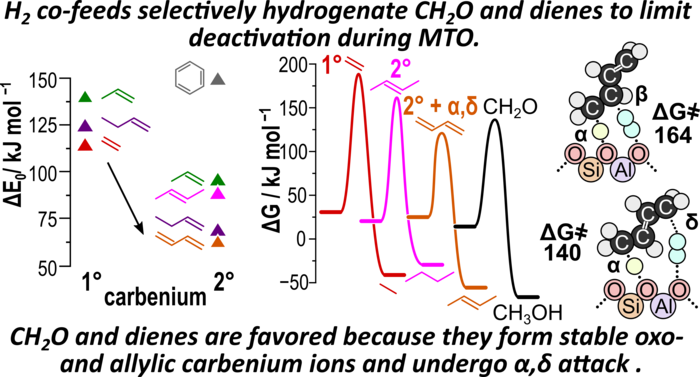
Abstract
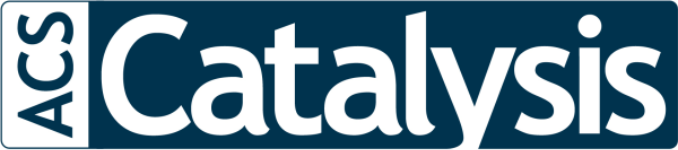
32. A. Almithn and D. Hibbitts*
“Impact of Metal and Heteroatom Identities in the Hydrogenolysis of C–X Bonds (X = C, N, O, S, and Cl)”
ACS Catalysis, 10 (2020) 5086–5100.
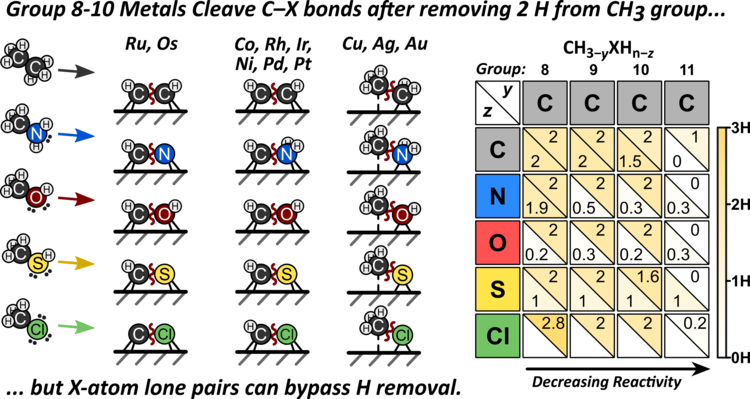
Abstract
2019
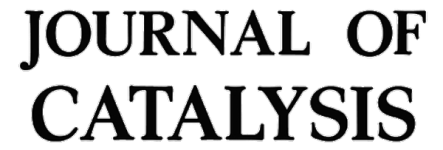
31. J. Di Iorio, A. Hoffman, C. Nimlos, S. Nystrom, D. Hibbitts*, and R. Gounder*
“Mechanistic Origins of the High-Pressure Inhibition of Methanol Dehydration Rates in Small-Pore Acidic Zeolites.”
Journal of Catalysis, 380 (2019) 161–177.
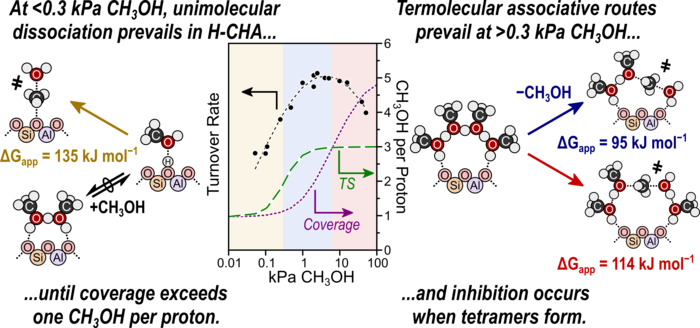
Abstract
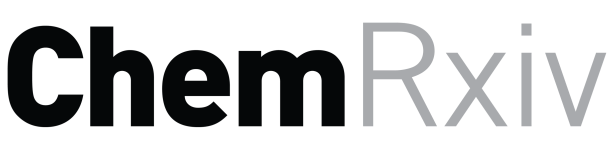
30. P. Kravchenko, C. Plaisance, and D. Hibbitts*,
“A New Computational Interface for Catalysis.”
Pre-print, (2019).
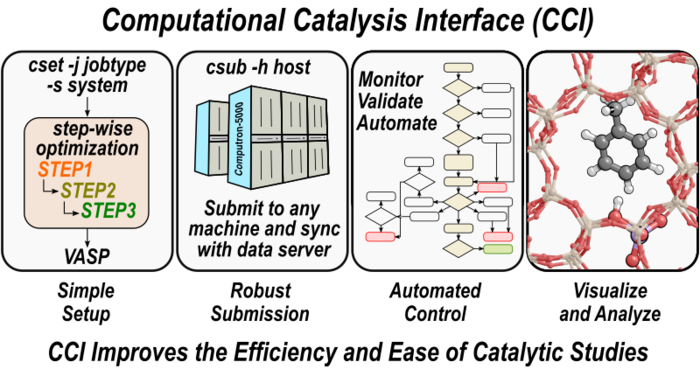
Abstract
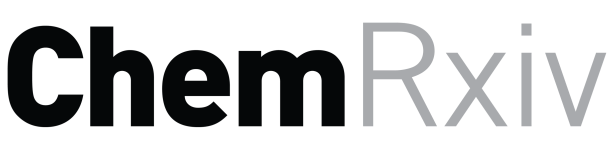
29. M. DeLuca and D. Hibbitts*,
“Prediction of C6–C12 Interconversion Rates Using Novel Zeolite-specific Kinetic Monte Carlo Simulation Methods.”
Pre-print, (2019).
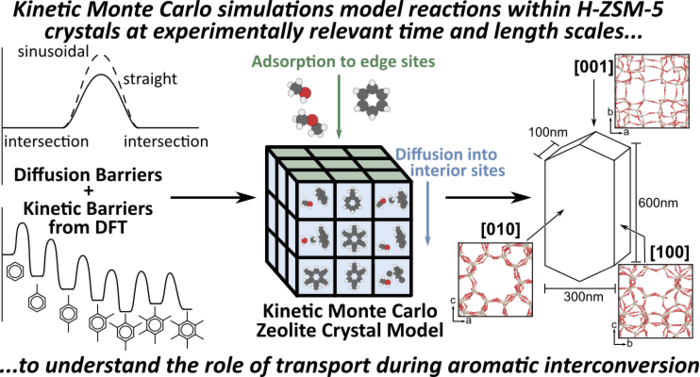
Abstract
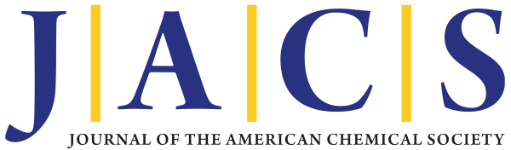
28. M. Witzke, A. Almithn, C. Coonrod, M. Triezenberg, D. Hibbitts*, and D. Flaherty*,
“In situ Spectroscopic Methods for Isolating Reactive Intermediate Structures during Hydrogenolysis Reactions.”
Journal of the American Chemical Society, 141 (2019) 16671–16684.
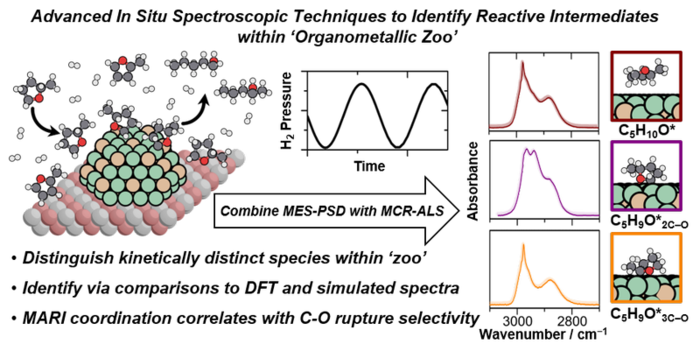
Abstract
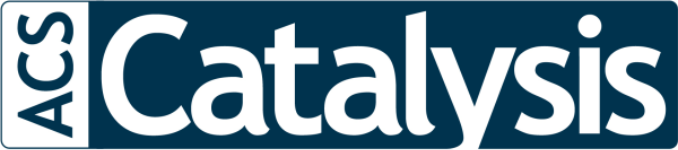
27. M. DeLuca, P. Kravchenko, A. Hoffman, and D. Hibbitts*,
“Mechanism and Kinetics of Methylating C6–C12 Methylbenzenes with Methanol and DME in H-MFI Zeolites.”
ACS Catalysis, 9 (2019) 6444–6460.
Front Cover Article!
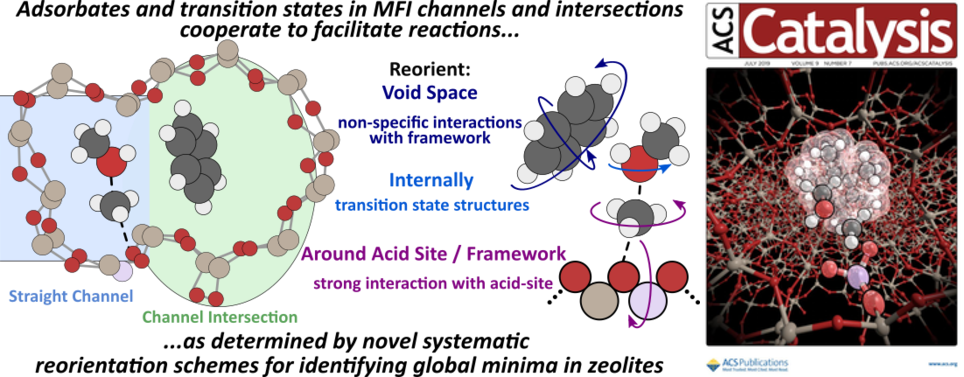
Abstract
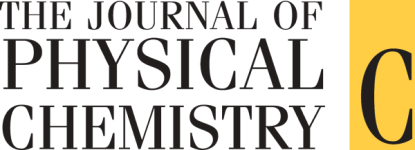
26. A. Hoffman, M. DeLuca, and D. Hibbitts*,
“Restructuring of MFI Framework Zeolite Models and their Associated Artifacts in Density Functional Theory Calculations.”
Journal of Physical Chemistry C, 123 (2019) 6572–6585.
Editor's Choice!
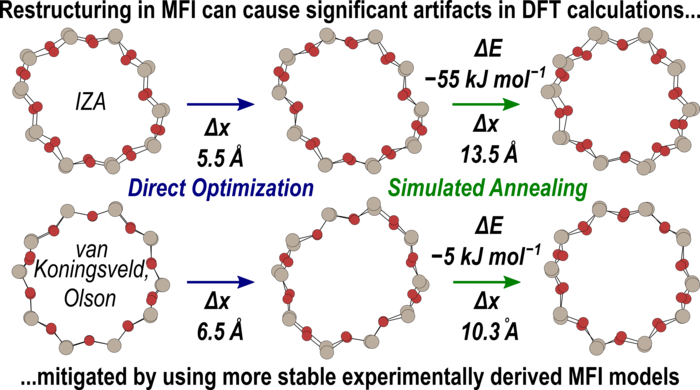
Abstract
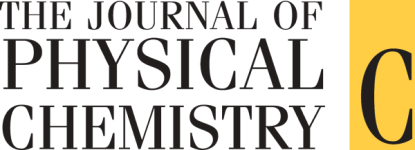
25. A. Almithn and D. Hibbitts*,
“Comparing Rate and Mechanism of Ethane Hydrogenolysis on Transition Metal Catalysts.”
Journal of Physical Chemistry C, 123 (2019) 5421–5432.
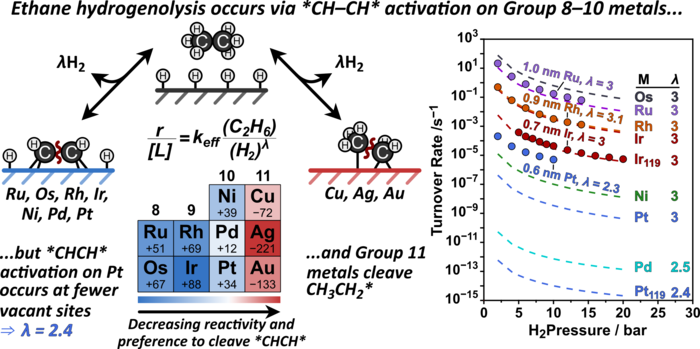
Abstract
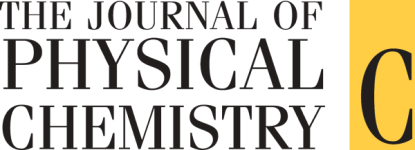
24. M. Garcia-Dieguez, D. Hibbitts*, and E. Iglesia*,
“Hydrogen Chemisorption Isotherms on Pt Particles at Catalytic Temperatures: Langmuir and Two-Dimensional Gas Models Revisited.”
Journal of Physical Chemistry C, 123, (2019), 8447–8462.
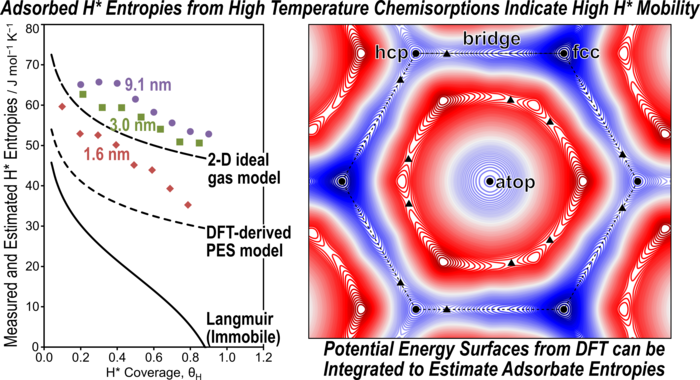
Abstract
2018
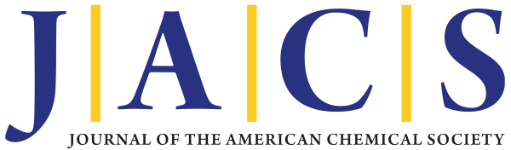
23. M. Cordon, J. Harris, J. Vega-Vila, J. Bates, S. Kaur, M. Gupta, M. Witzke, E. Wegener, J. Miller, D. Flaherty, D. Hibbitts, R. Gounder*,
“The Dominant Role of Entropy in Stabilizing Sugar Isomerization Transition States within Hydrophobic Zeolite Pores.”
Journal of the American Chemical Society, 140 (2018) 14244–14266.
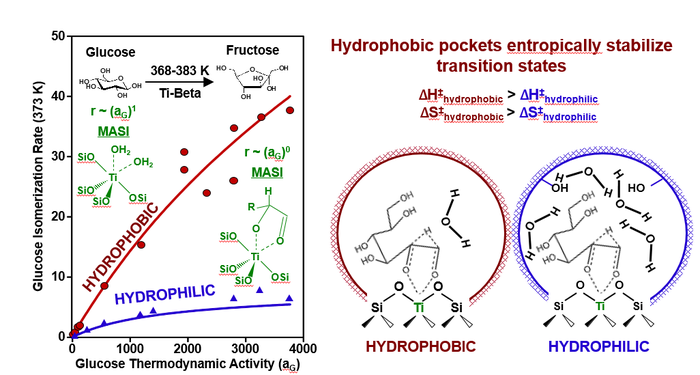
Abstract
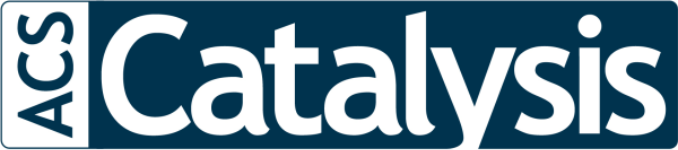
22. S. Nystrom^, A. Hoffman^, and D. Hibbitts*,
“Tuning Brønsted Acid Strength by Altering Site Proximity in CHA Framework Zeolites.”
ACS Catalysis, 8 (2018) 7842–7860.
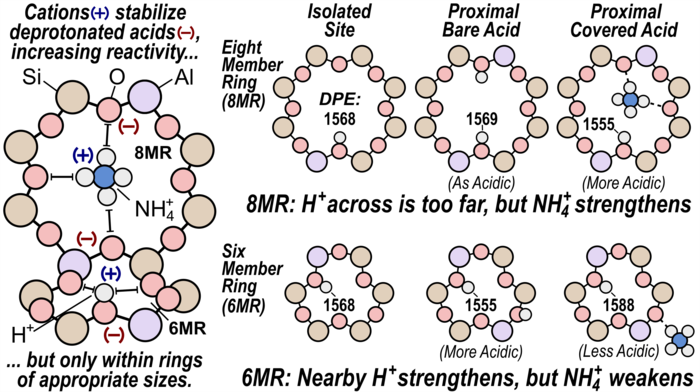
Abstract
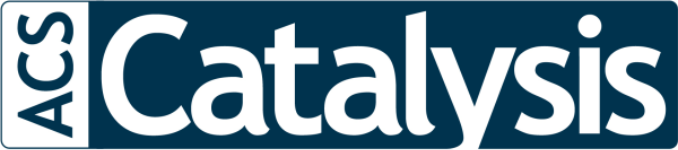
21. M. Witzke, A. Almithn, C. Coonrod, D. Hibbitts*, and D. Flaherty*,
“Mechanisms and Active Sites for C-O Bond Rupture within 2-Methyltetrahydrofuran over Nickel Phosphide Catalysts.”
ACS Catalysis, 8 (2018) 7141–7157.

Abstract
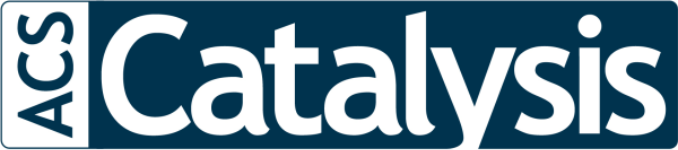
20. A. Almithn and D. Hibbitts*,
“Effects of Catalyst Model and High Adsorbate Coverages in ab initio Studies of Alkane Hydrogenolysis.”
ACS Catalysis, 8 (2018) 6375–6387.
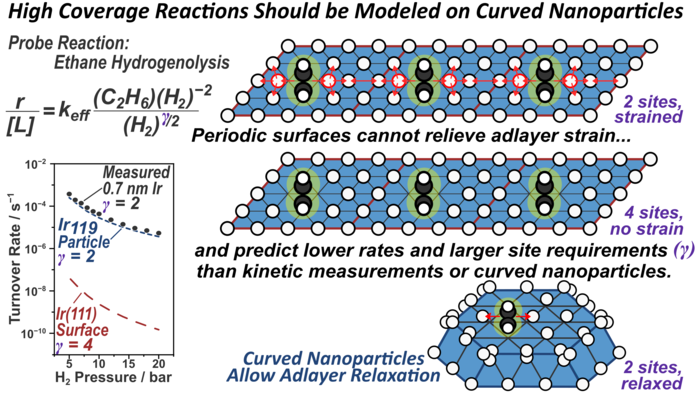
Abstract
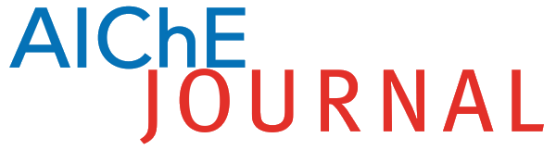
19. A. Almithn and D. Hibbitts*,
“Supra-Monolayer Coverages on Small Metal Clusters and Their Effects on H2 Chemisorption Particle Size Estimates.”
AIChE Journal, 64 (2018) 3109–3120. Invited.
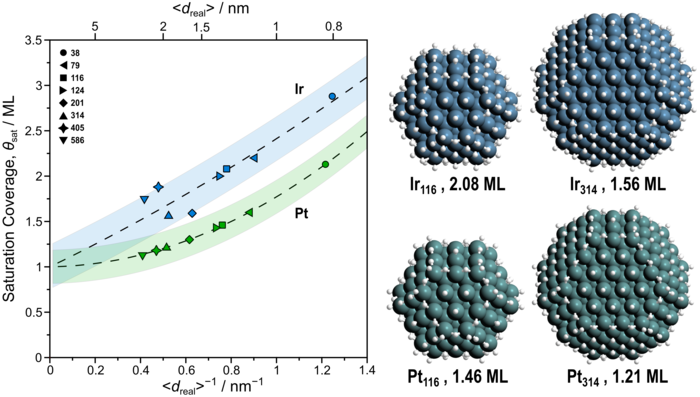
Abstract
2017
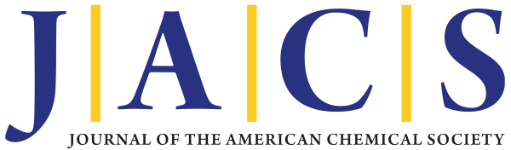
18. J. Liu, D. Hibbitts*, and E. Iglesia*,
“Dense CO Adlayers as Enablers of CO Hydrogenation Turnovers on Ru Surfaces.”
Journal of the American Chemical Society, 139 (2017) 11789–11802.
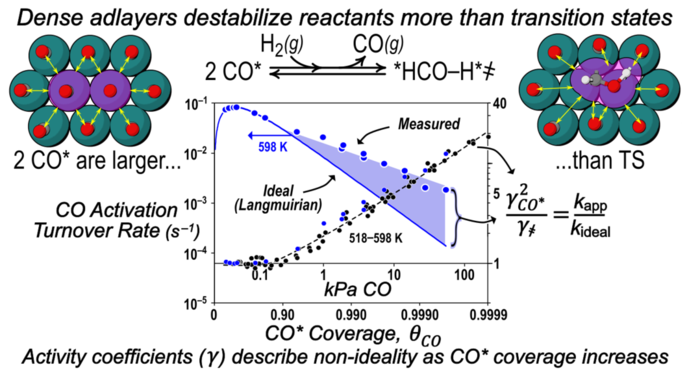
Abstract
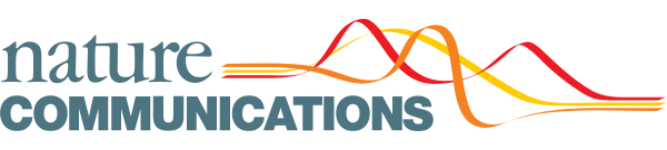
17. R. Rao, R. Blume, T, Hansen, E. Fuentes, K. Dreyer, S. Moldovan, O. Ersen, D. Hibbitts, Y. Chabal, R. Schlogl, and J. Tessonnier*,
“Interfacial charge distributions in carbon-supported palladium catalysts.”
Nature Communications, 8 (2017) 340:1–10.
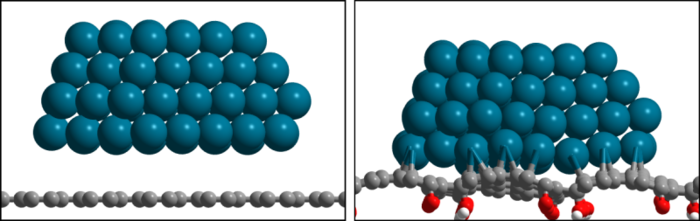
Abstract
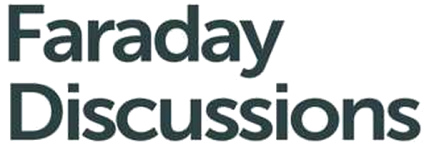
16. M. Neurock*, Z. Tao, A. Chemburkar, D. Hibbitts, and E. Iglesia,
“Theoretical Insights into the Sites and Mechanisms for Base Catalyzed Esterification and Aldol Condensation Reactions over Cu.”
Faraday Discussions, 197 (2017) 59–86. Invited.
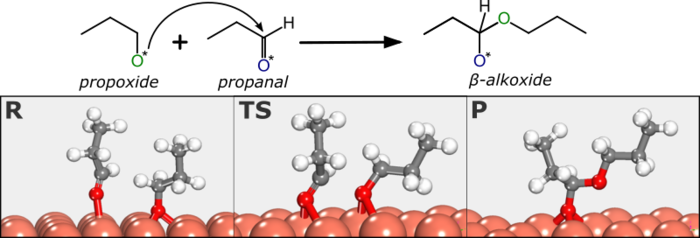
Abstract
2016
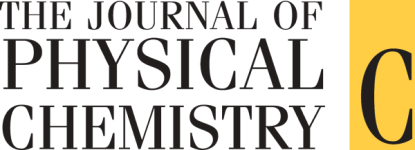
15. D. Hibbitts, D. Flaherty, and E. Iglesia*,
“Effects of Chain Length on the Mechanism and Rates of Metal-Catalyzed Hydrogenolysis of n-Alkanes.”
Journal of Physical Chemistry C, 120 (2016) 8125–8138.
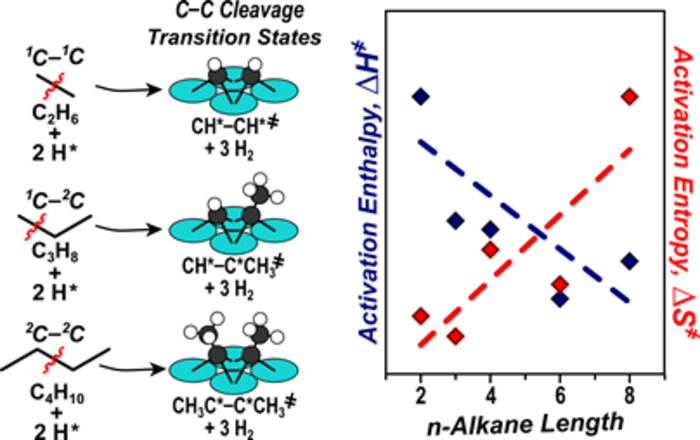
Abstract
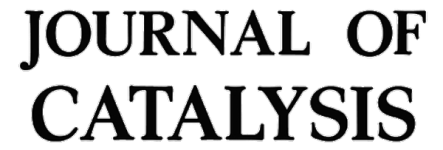
14. D. Hibbitts, E. Dybeck, T. Lawlor, M. Neurock*, and E. Iglesia*,
“Preferential Activation of Carbon Monoxide near Hydrocarbon Chains during Fischer-Tropsch Synthesis.”
Journal of Catalysis, 337 (2016) 91–101.
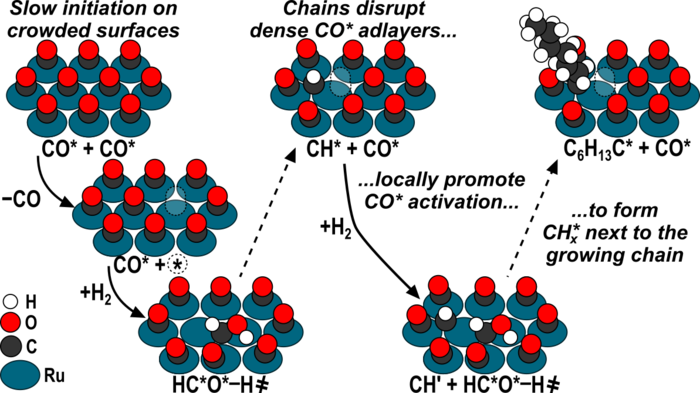
Abstract
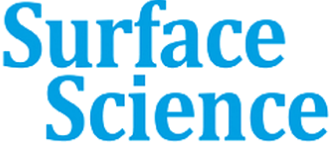
13. D. Hibbitts and M. Neurock*,
“Promotional Effects of Chemisorbed Oxygen and Hydroxide in the Activation of C–H and O–H Bonds on Transition Metal Surfaces.”
Surface Science, 650 (2016) 210–220.
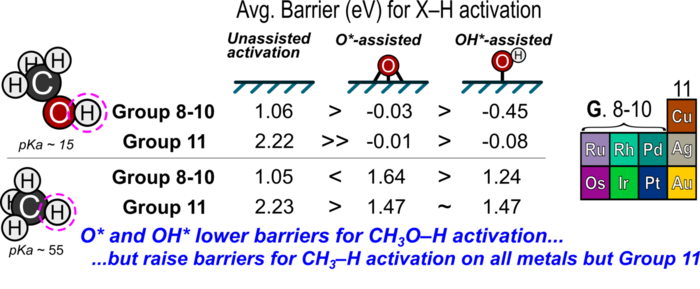
Abstract
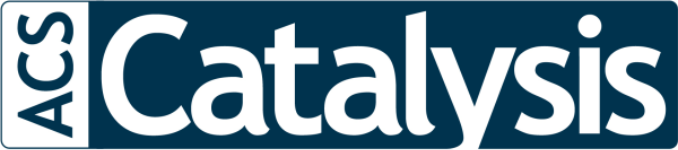
12. D. Hibbitts, D. Flaherty, and E. Iglesia*,
“Role of Branching on the Rate and Mechanism of C–C Cleavage in Alkanes on Metal Surfaces.”
ACS Catalysis, 6 (2016) 469–482.
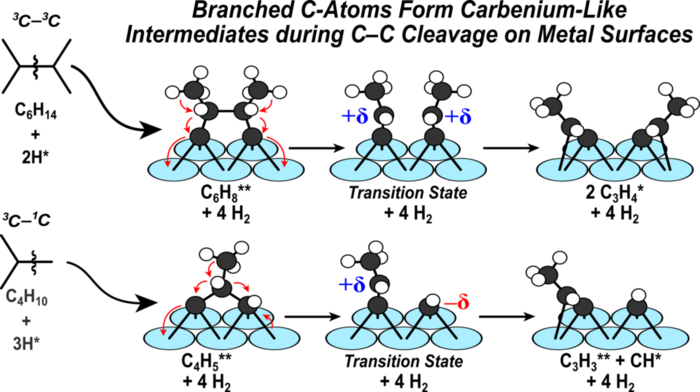
Abstract
2015
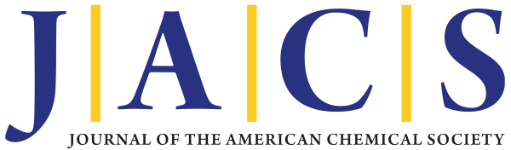
11. E. Gurbuz, D. Hibbitts, and E. Iglesia*,
“Kinetic and Mechanistic Assessment of Alkanol/Alkanal Decarbonylation and Deoxygenation Pathways on Metal Catalysts.”
Journal of the American Chemical Society, 137 (2015) 11984–11995.
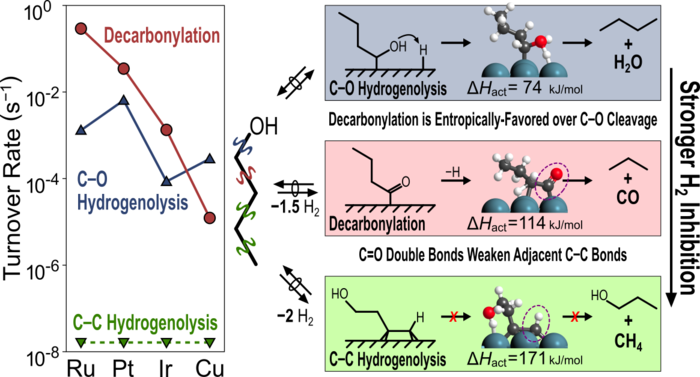
Abstract
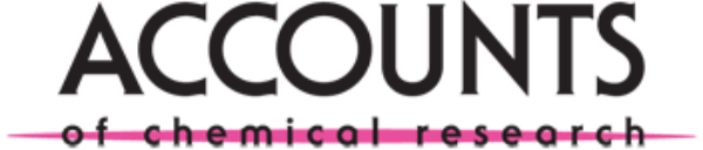
10. D. Hibbitts and E. Iglesia*,
“The Prevalence of Bimolecular Routes in the Activation of Diatomic Molecules with Strong Chemical Bonds (O2, NO, CO, N2) on Catalytic Surfaces.”
Accounts of Chemical Research, 48 (2015) 1254–1262.
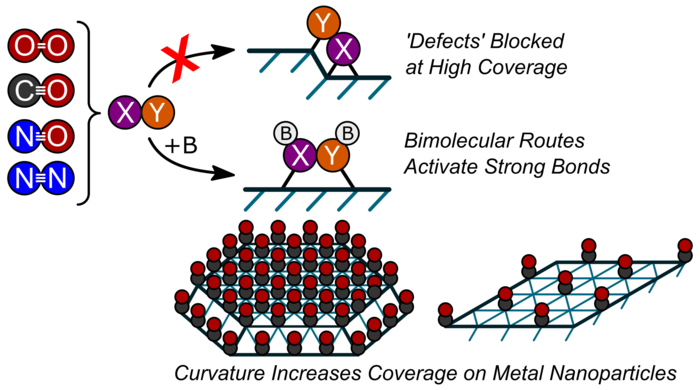
Abstract
2014
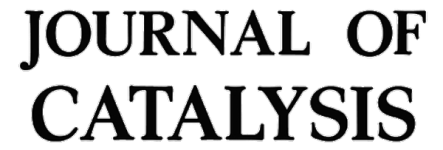
9. D. Hibbitts, R. Jimenez, M. Yoshimura, B. Weiss, and E. Iglesia*,
“Catalytic NO activation and NO‑H2 Reaction Pathways.”
Journal of Catalysis, 319 (2014) 95–109.
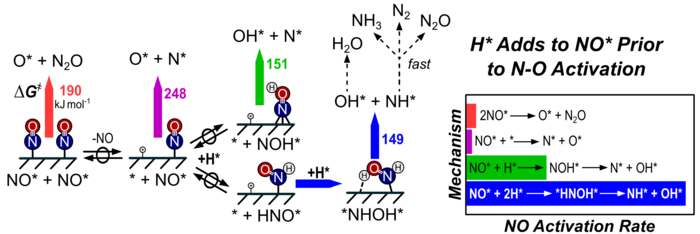
Abstract
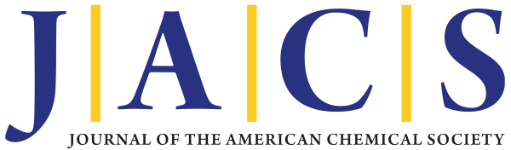
8. D. Flaherty, D. Hibbitts, and E. Iglesia*,
“Metal-Catalyzed C–C Bond Cleavage in Alkanes: Effects of Methyl Substitution on Transition State Structures and Stability.”
Journal of the American Chemical Society, 136 (2014) 9664–9676.
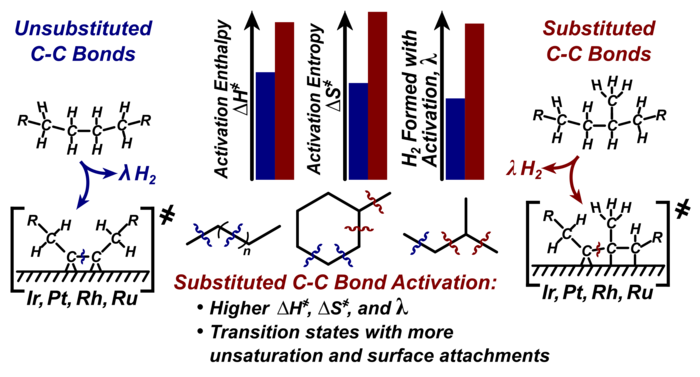
Abstract
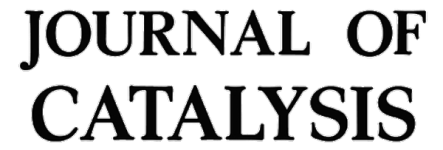
7. D. Hibbitts, Q. Tan, and M. Neurock*,
“Acid Strength and Bifunctional Catalytic Behavior of Alloys Comprised of Noble Metals and Oxophilic Metal Promoters.”
Journal of Catalysis, 315 (2014) 48–58.
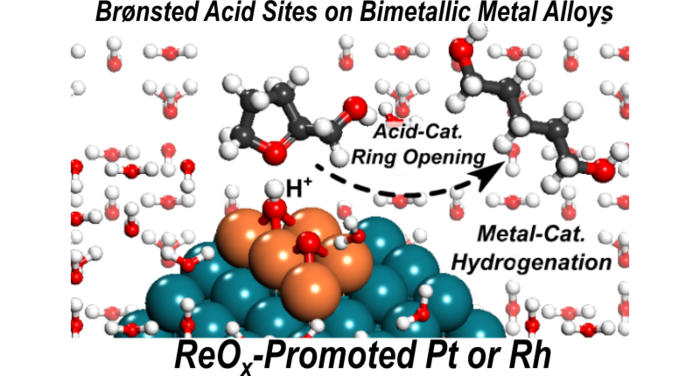
Abstract
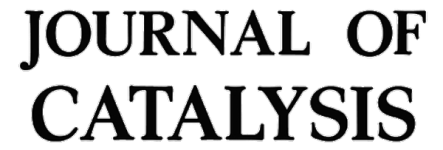
6. D. Flaherty^, D. Hibbitts^, E. Gurbuz, and E. Iglesia*,
“Theoretical and Kinetic Assessment of the Mechanism of Ethane Hydrogenolysis on Metal Surfaces Saturated with Chemisorbed Hydrogen.”
Journal of Catalysis, 311 (2014) 350–356.
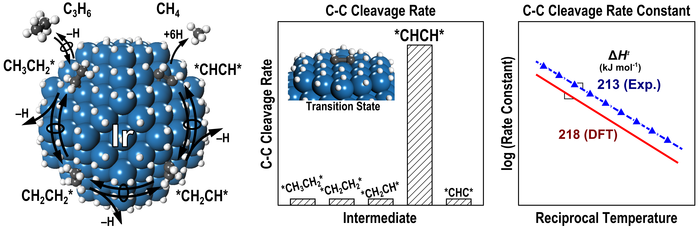
Abstract
2013
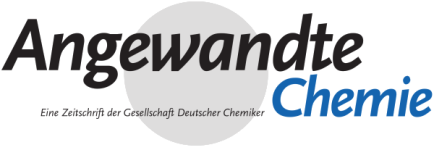
5. D. Hibbitts, B. Loveless, M. Neurock, and E. Iglesia*,
“Mechanistic Role of Water on the Rate and Selectivity of Fischer-Tropsch Synthesis on Ruthenium Catalysts.”
Angewandte Chemie, 52 (2013) 12273–12278.
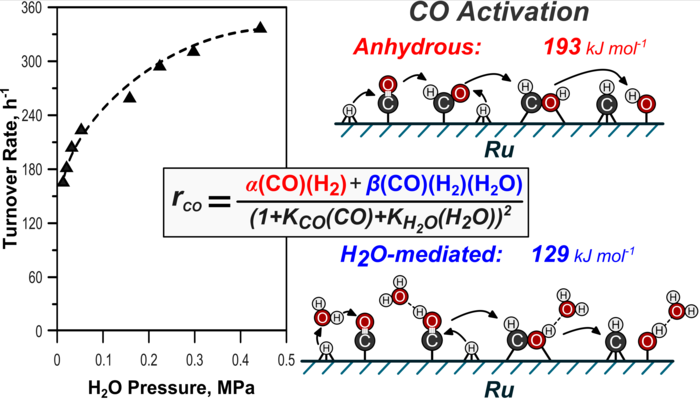
Abstract
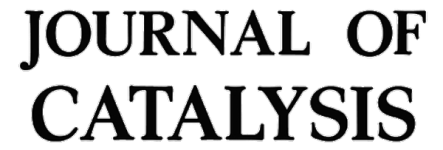
4. D. Hibbitts and M. Neurock*,
“Influence of Oxygen and pH on the Selective Oxidation of Ethanol on Pd catalysts.”
Journal of Catalysis, 299 (2013) 261–271.
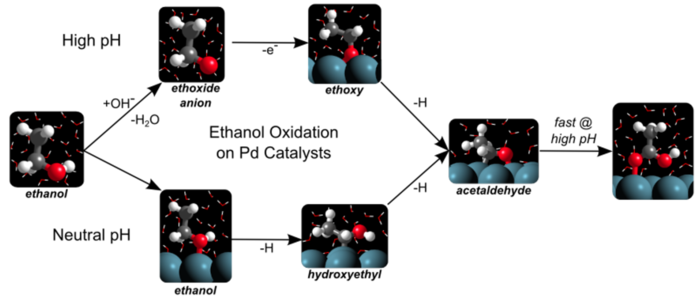
Abstract
2012
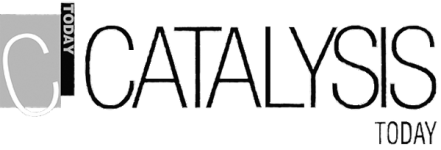
3. B. Braunchweig, D. Hibbitts, M. Neurock, and A. Wieckowski*,
“Electrocatalysis: a Fuel Cell and Surface Science Perspective.”
Catalysis Today, 202 (2013) 197–209.
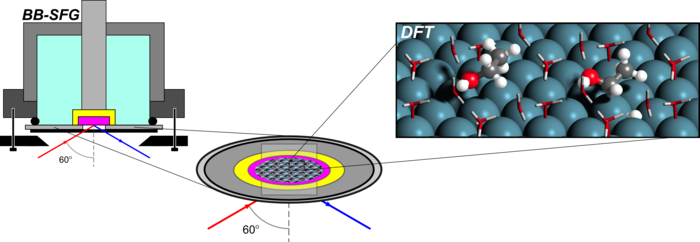
Abstract
2011
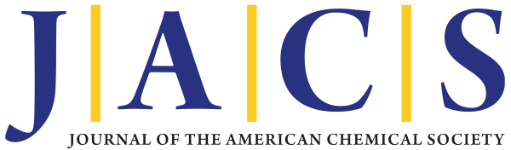
2. M. Chia, Y. Pagan-Torres, D. Hibbitts, Q. Tan, H. Pham, A. Datye, M. Neurock, R. Davis, and J. Dumesic*,
“Selective Hydrogenolysis of Polyols and Cyclic Ethers over Bi-Functional Surface Sites on Rhodium-Rhenium Catalysts.”
Journal of the American Chemical Society, 133 (2011) 12675–12689.
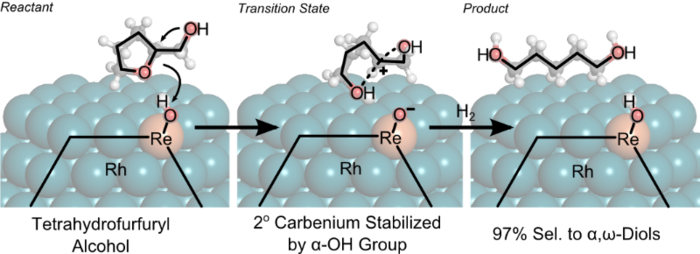